Published on February 2, 2024
To enable production volumes of green hydrogen to ramp up, meeting the water demand necessary to produce it is crucial. Green hydrogen from renewable sources is produced through electrolysis, a process that requires large amounts of clean water. Green hydrogen’s resource-intensive production process is often the focus of attention, especially as regions with limited water resources have the highest potential for its production. Most areas with high renewable energy potential from sun and wind are advantageous for efficient hydrogen production, but are also classified as water-scarce regions. Therefore, in many regions, desalination emerges as a necessary solution. It can also provide co-benefits beyond hydrogen production by contributing to the improvement of water supply for local communities and businesses. Water desalination therefore emerges as a crucial, perhaps even necessary, solution in these regions.
Seawater desalination is an established technology: 16,800 desalination plants in 150 countries provide fresh water to some 300 million people worldwide [1]. However, it is important to recognise that while desalination is a promising technology, it is not a silver bullet, as it poses environmental challenges if not managed properly. The energy intensity of the desalination process and the disposal of brine, a high-salinity by-product, are key challenges. The energy needed for a desalination process relies on factors such as the quality of the input water, the extent of water treatment, the technology employed by the facility, and the capacity of the plant [3]. Table 1 displays how much energy the desalination of 1 m3 of drinking water requires [3].
Water source | Energy (kWh/m3) |
Surface water (lake or river) | 0.37 |
Groundwater | 0.48 |
Wastewater treatment | 0.62 – 0.87 |
Wastewater reuse | 1.0 – 2.5 |
Seawater | 2.58 – 8.5 |
Brine contains not only high concentrations of salt, but also chemicals that can affect the environment. Effective brine management is therefore essential to minimise environmental impact.
The real water demand
The distinction between water consumption and water extraction plays a crucial role in hydrogen production. Technically speaking, the expected requirement for producing 1 kg of hydrogen is about 9 to 11 litres of fresh water. However, the figure is misleading because the actual amount of water used is much higher.
Figure 1 shows that one kilogramme of hydrogen requires 35 kg of desalinated water [5]. This is mainly due to the fact that a considerable amount of water is required for the electrolysis cooling process. The water consumption for cooling is determined by the specific design of the cooling solution. The most conventional cooling methods are based on dry or wet cooling, although hybrid solutions are also available. With dry cooling, large radiator and fan arrays are used to dissipate the heat from the electrolyser into the ambient air. Therefore, no additional water is required. However, dry cooling generally has higher energy costs for the same amount of heat than wet cooling and the investment costs are higher. In addition, this type of cooling is less efficient in hot and dry climates. In wet cooling, water is used to cool the electrolyser, with varying levels of water consumption. Therefore, the supply of additional desalinated water is required. However, this method generally has lower investment costs and lower energy costs than dry cooling. In the example below, water-based cooling is considered.
To desalinate 35 kg of water, 83 kg of seawater must be extracted, resulting in the production of 48 kg of brine as a by-product. The conversion of the input water into high-quality water depends primarily on the water recovery ratio of the specific process (reverse osmosis in this case) and the quality of the input water.
Taking into account the above-mentioned points, the production of 1 kg of hydrogen results in a mixture of 59 kg, mainly consisting of brine and cooling water.
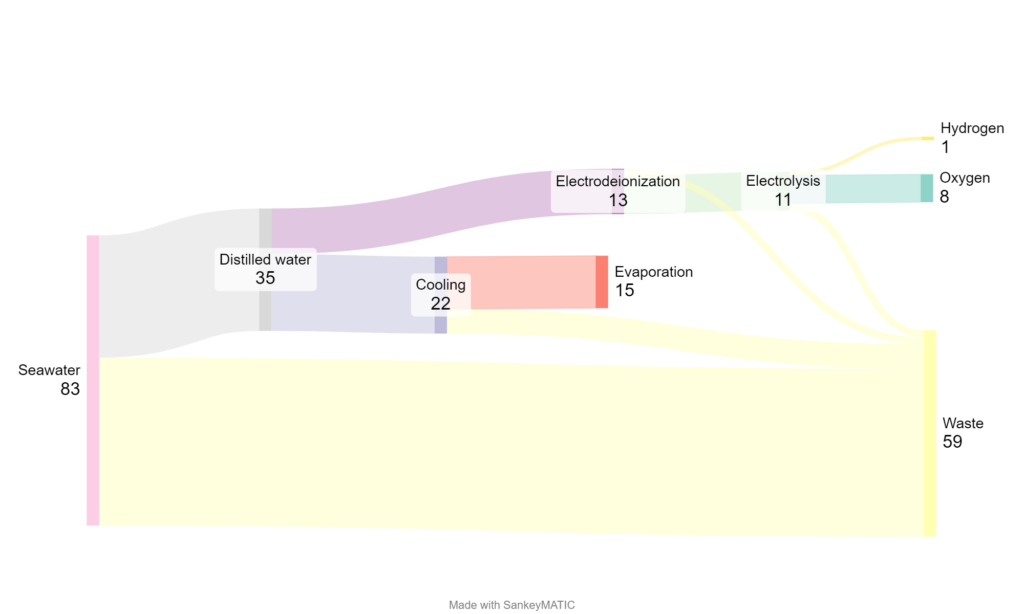
Challenges of brine discharge back into the sea
Discharging wastewater, especially brine, into the sea has significant environmental drawbacks. A primary concern is the increase in salinity of seawater, which can have profound effects on the marine ecosystem. Increased salinity and reduced dissolved oxygen content can have ecological consequences throughout the marine food chain.
Discharging brine into the sea also increases the concentration of heavy metals. The liquid wastewater concentrate from a desalination plant contains a high proportion of soluble salts and metals such as copper, cadmium, lead, mercury, nickel, chromium and arsenic. These heavy metals in desalination concentrates can exceed discharge limits and have a negative ecological impact on marine life.
In addition, toxic chemicals such as copper and chlorine are used to protect the membranes of the desalination process against lime scale and bacterial growth. As a result, the brine also contains copper and chlorine.
Brine remains one of the most significant barriers to the sustainable and widespread adoption of desalination technologies. The challenge is to find solutions that increase freshwater production, minimise environmental impact, and enable the sustainable management of the waste produced.
Solutions on the way
One promising approach is to invest in brine treatment facilities that aim to reduce environmental impact by avoiding conventional discharge methods. These projects avoid the direct discharge of brine into the sea. Instead, the brine is extracted from the desalination plant in solid form and used in a highly concentrated stream for further processing steps. These are standard chemical processes that are used to convert the brine. Initially, nanofiltration, a pressure-driven membrane process that separates the smallest molecules from a solution is used to remove unwanted compounds. Next, one or more electrodialysis steps (process for transporting salts from one solution to another) are used to produce the desired end product [2]. These are not new procedures, as researchers have analysed the potential for producing useful chemicals from brine. They could be used commercially to improve the economics of the desalination process.
This innovative approach not only minimises potential negative effects on the marine ecosystem, but also allows for more efficient use of the waste products. By extracting minerals and metals from the brine, there is an opportunity to reuse these materials in a sustainable cycle. This approach can for example be used for producing sodium hydroxide (NaOH). NaOH can be utilised for pretreating seawater entering the desalination plant. This alters the water’s acidity, helping to prevent membrane fouling, one of the main causes of disruptions and failures in existing desalination plants [2].
Desalination technology stands as a promising solution to address water scarcity in numerous developing countries. Despite its potential, there are persistent challenges that necessitate advancements in technology and regulatory support. One crucial aspect is the need for policymakers to regulate the disposal of brine. Considering local circumstances, the evaluation of factors such as the composition and quantity of chemicals, brine temperature, salinity content, and the overall volume of disposed brine is essential. Implementing appropriate brine management measures based on these assessments is crucial. Also, desalination has experienced significant technological innovations. Breakthroughs like nanostructured membranes, and the development of new energy recovery devices, which for example help to recover energy from the high-pressure brine stream that is discharged from the reverse osmosis membranes, are anticipated to enhance productivity and reduce energy costs by over 30% [4].
Interested in the role of water and other sustainability aspects in PtX value chains? Check out our six briefings discussing key elements of PtX sustainability for ensuring long-lasting positive impacts for all stakeholders directly or indirectly involved. The briefing on water desalination can be downloaded here. The other briefings are available in our Knowledge Base.
Sources:
[1] Eke J., Yusuf, A., Giaw, A., Sodiq, A. (2020): The global status of desalination: An assessment of current desalination technologies, plants and capacity
[2] David L. Chandler (2019): Turning desalination waste into a useful resource. Massachusetts Institute of Technology
[3] Nassrullah H., Anis S. Hashaikeh R. Hilal N. (2020) Energy for desalination: A state-of-the-art review. Centre for Water Advanced Technologies and Environmental Research (CWATER)
[4] A. Al Amoudi ,N. Voutchkov (2021). Innovation in Desalination – The Path Forward
[5] Jaeger, P., & Salgado, T. (2023). Desalinización de Agua de Mar y Reutalización de Aguas de Desecho para la Producción de Hidrógeno Verde y Derivados en Chile. International PtX Hub